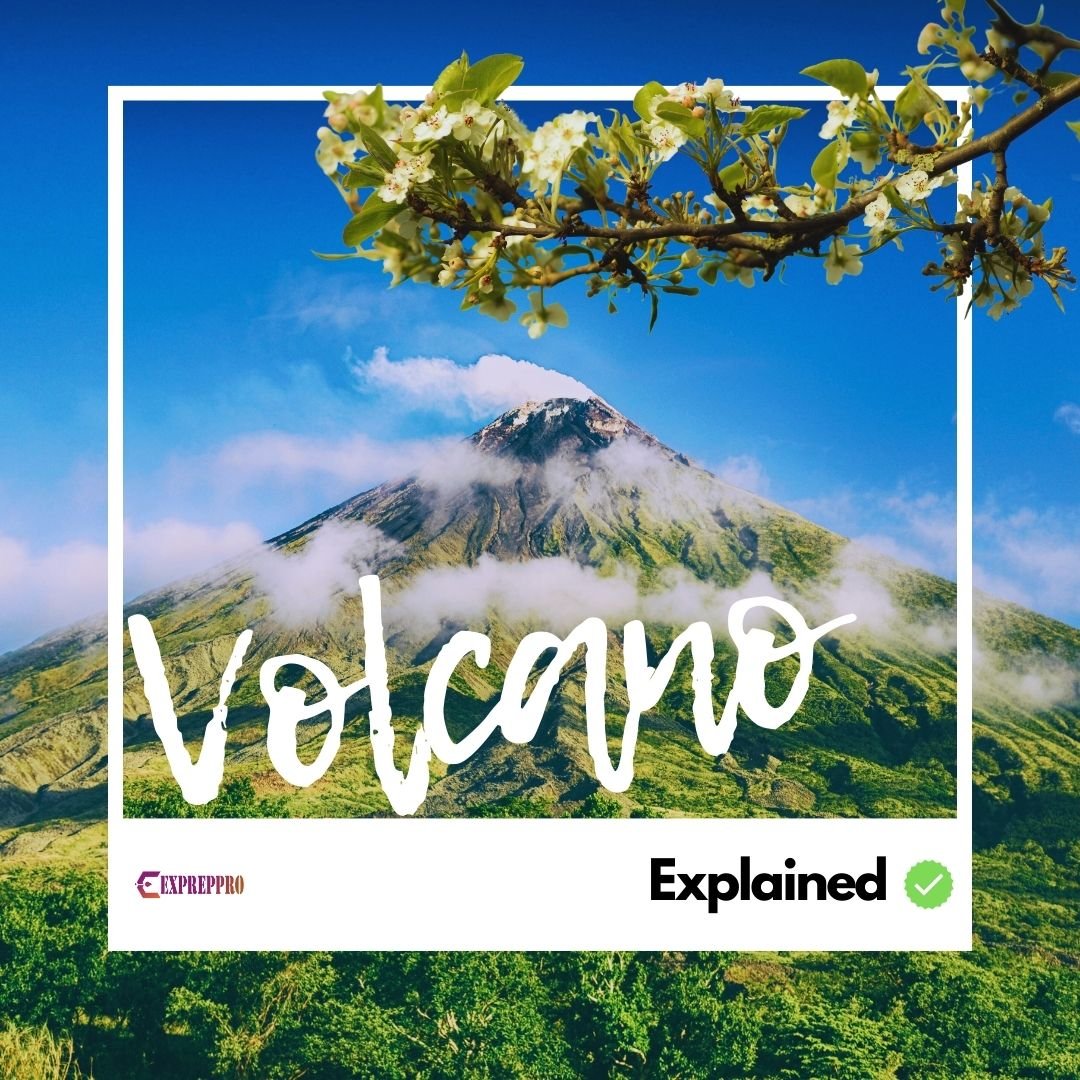
The volcano is a vent or opening through which molten materials and gases are ejected from the interior to the surface of the Earth. You may have noticed this spectacular and dangerous phenomenon in newspapers, books, magazines, and videos. The wrath of this natural phenomenon can result in long-term as well as short-term effects on the lives of humans. Volcanoes can even influence the lives of people who live thousands of kilometres away. For instance, when Indonesia’s Mount Ruang volcano erupted in April 2024, the tower of volcano ash reached more than five kilometres into the sky. This event prompted air traffic to close airspace and Sam Ratulangi International Airport, which is more than 100 kilometres from Mount Ruang.
MAGMA OR LAVA
What is the difference between Magma and Lava? Most of us use these words as synonyms.
But there is a difference. Molten rock in the Earth’s deep interior is known as Magma, and When Magma reaches the surface, it is called lava rather than Magma.
ORIGIN OF MAGMA
Magma is formed by the melting of rocks. Besides the liquid core, the interior of Earth consists of solid rocks, and in some parts of the interior, the rocks have a gummy consistency. Technically, they are all considered solid. So, the Magma is produced locally when specific conditions are met. In most cases, the driving force is plate tectonics. That’s why most volcanoes occur in the plate boundaries or at developing plate boundaries. But there is an exception: Mantle plume. The mantle plume appears in the plate’s interior, on land, and in the ocean.
Other factors besides heat, like pressure, composition, and volatile content, can result in hot rocks melting. However, for these factors to be relevant, heat is required.
There are four primary heat sources active inside the Earth: Nebulkar, Kinetic, Gravitational, and Radiogenic. Nebular and Kinetic energy were significant during the Earth’s initial formation period. Later, gravitational and nebular energy replaced them as the major contributors to Earth’s heat.
INTERNAL STRUCTURE OF EARTH
The present-day internal structure evolved due to the subsequent modification by physical and chemical processes such as heating, convective circulation, and geothermal differentiation.
The Earth’s interior consists of three concentric shells of different composition and density: the crust, the mantle, and the core. This interpretation is based on multiple lines of evidence, such as a sharp break in density with an increase in depth, Changes in the velocity of the P-wave and S-wave, and Comparisons with the meteorite composition spectrum.
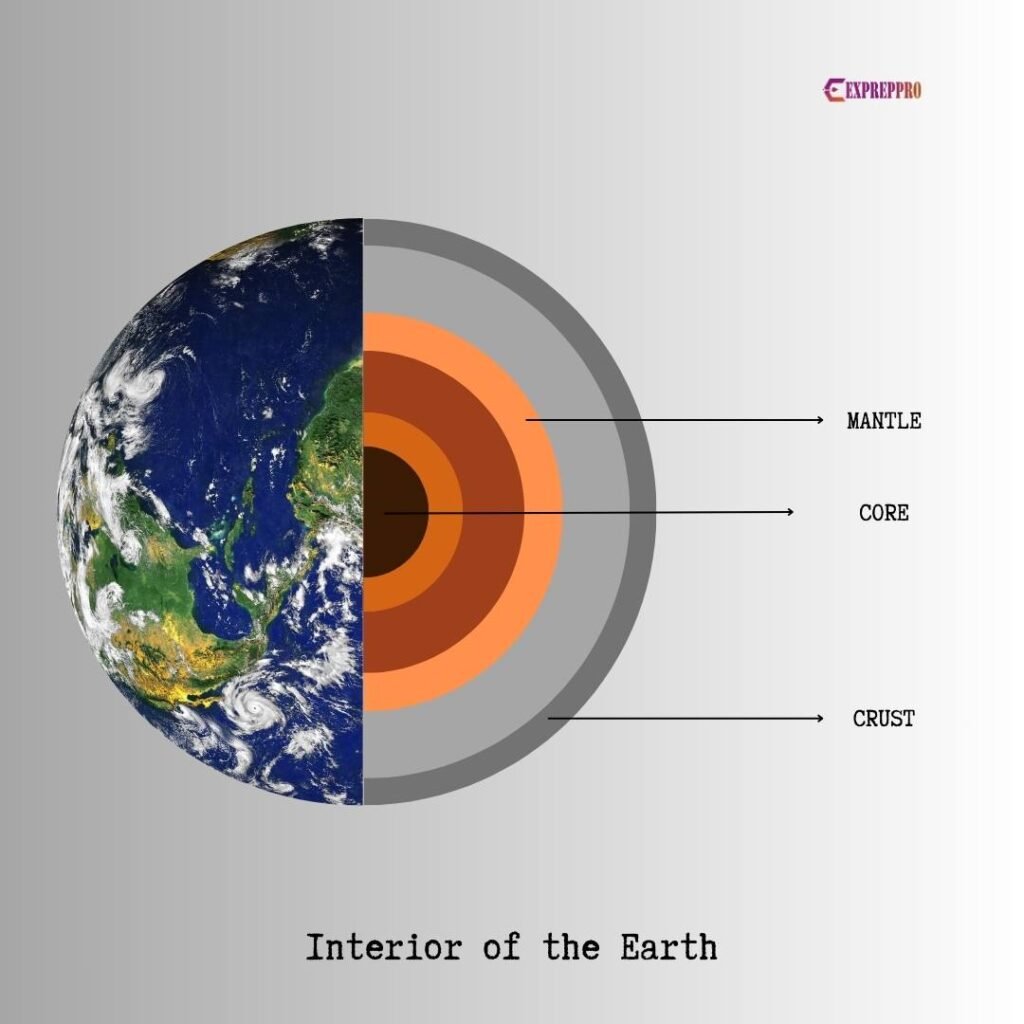
THE EARTH CRUST
The crust is the outermost thin layer of the Earth. It is volumetrically insignificant because it forms only zero point seven per cent of the Earth’s mass. This portion of Earth is significant for living beings. The crust is composed of almost Silicate materials, and eight elements: Oxygen, Silicon, Aluminium, Iron, Calcium, Magnesium, Sodium, and Potassium form up to 99 per cent of the total.
There are two different types of crust. The first one is the Oceanic crust that lies below the ocean, covering around 70 per cent of the Earth’s surface area. The average thickness is seven kilometres. In some regions with abundant basaltic lava outpourings, the thickness may reach a level of 10 km. The oceanic crust is composed of relatively dense “mafic” rocks. Mafic rocks are formed of silicate minerals and are rich in Iron and Magnesium. These rocks are relatively denser and dark in colour.
Continental crust
The second one is the continental crust. The continental crust is more complex, topographically higher, much older, thicker and has rocks with high silica and aluminium or sial. They have a higher amount of silica, with many regions having relatively high concentrations of quartz. We can also notice “Andestic” composition or rocks enriched in silicon, sodium, potassium, volatiles and radioactive elements.
The concentration of quartz in the crust is important. In the presence of a small amount of water, the quartz-rich rock becomes relatively flexible at mid-temperature and pressure. The density of the continental crust is low compared to that of the oceanic crust. The continental crust density is 2500 kilograms per cubic meter, but the oceanic crust density is 3000 kilograms per cubic meter. The crust and upper part of the mantle form the lithosphere with rigid, brittle rocks. The thickness ranges between 60 to 150 kilometres, which is thin under the ocean crust and thick under the continental crust.
The lithosphere floats on a gummy or plastic Asthenosphere, much like an ice that floats in water. Naturally, the weight will sink until it reaches its buoyancy point. Buoyancy is the tendency of an object to float when submerged. Once the buoyancy point is reached, it is supported by the Asthenosphere. It is in a state of equilibrium called isostasy.
Isostatic rebound
Once the weight changes, the lithosphere will rise. It is usually measured in thousands of years, and erosion reduces the height and weight of a mountain over time, resulting in a reduction in weight and rise back up over geological time. This phenomenon is known as isostatic rebound. The case of the Adirondack Mountains is an example. These mountains experience the isostatic rebound phenomenon. The Asthenosphere is partially molten because the temperature is around 1100 degrees Celsius at a depth of 100 kilometres. This 100-kilometre is supposed to be nearer to the initial melting point.
The Earth’s crust is separated from the mantle by a discontinuity called the Mohorovic discontinuity. It is named after the Croatian geologist Andrija Mohorovic, who proposed its existence. The sudden change in seismic waves due to density change helped him identify the Moho discontinuity. Below the continents, the Moho discontinuity averages around 35 kilometres, and it is about 7 kilometres below the ocean floor.
The presence of a chemically evolved continental crust is a unique feature of our planet, Earth, compared to other rocky planets in our solar system.
The boundary between the lithosphere and Asthenosphere is located at a depth of around 100 to 220 kilometres, where both P and S waves demonstrate a decrease in velocity and high electrical conductivity. This boundary is known as a Low-velocity zone, L.V.Z, or Lehmann discontinuity.
THE MANTLE
The mantle is present below the Earth’s crust, representing about 68 per cent of the mass and 83 per cent of the volume. It is divided into three regions based on major discontinuities in seismic velocities. The mantle is the layer found between the core and the crust, which is about 2900 kilometres thick.
It comprises mafic or magnesium-ferric, silicate minerals, and depleted volatile and radioactive elements. The upper mantle extends from the Mohorovic discontinuity to a depth of a thousand kilometres. Here, both temperature and pressure are low, so the rocks are less rigid. This softer, plastic part of the mantle is the Asthenosphere. This region of the Asthenosphere is a low-velocity zone in which seismic waves are strongly attenuated, indicating the possible presence of a semi-melt phase.
The second seismic discontinuity occurs at a depth of 650 to 670 kilometres; this region is called the transition zone. The third layer, or lower layer, is extended to 2900 kilometres deep. The temperature at the junction of the mantle and outer molten core standing at a depth of 2900 kilometres is about 2900 degrees Celsius. Even though the lower mantle is hotter than the upper mantle, it is largely rigid due to the intense pressure at the depth.
THE CORE
The core is the innermost layer located at the Earth’s centre and is about 3500 kilometres. It comprises about 16 per cent of the Earth’s volume and 31 per cent of the Earth’s mass. From density calculations, the core must be made of high-density elements. Seismic data also support this finding. If we assume chondritic meteorites to be the building blocks of terrestrial planets. In that case, the much lower concentrations of siderophile elements in the Earth’s upper mantle imply that these elements reside mainly in the core. The inner core is assumed to be molten due to 5000 degrees Celsius.
The low viscosity and inability to s-waves support this assumption. The inner core is solid, and the crystals probably exhibit a significant degree of common orientation. The outer liquid core can be rationalised by the disposition of the melting curve for metallic Iron and minor nickel relative to the Earth’s present geothermal gradient. Earth’s magnetic field originates from the differential motion between the outer core and the base of the solid, silicate mantle.
LITTLE HISTORY
Before learning more about volcanoes, let’s learn some history. There are many myths and legends about volcanoes. Myths have little utility for geoscientists, but legends help a lot. Traditional knowledge allows us to know various volcanoes better. Mount St. Helens in Washington has erupted many times in the past 4000 years. The first well-recorded eruption in the modern period was in 1980. However, the legends of Native Americans gave rich details about the eruptions that happened in the old period. The indigenous Mapuche community of Chile and Patagonia resides in a place with many active volcanoes. Their history and oral legends helped geoscientists.
The ancient Greeks believed that Vulcan, the god of the underworld and artisans, was responsible for volcanic activity, and the name volcano was derived from the god’s name. But when the period of the Renaissance started, people started to seek more logical reasons to explain volcanic activities. But the result was stiff opposition from conservative persons.
The Geognosic theory of geology and the Neptunist school
Abraham Gottloh Werner, a German professor of mineralogy from Freiburg, introduced a new theory, the Geognosic theory of geology. This theory was based on the biblical interpretation, so conservative authorities widely embraced it. He pointed out that the Earth had cooled solid long ago but had later been convened by a primitive, global ocean from which all stratified rocks and minerals in the crust precipitated. Fossils in some strata, though absent in lava flows, were good evidence of marine origin. Volcanoes were merely anomalies associated with burning coal deposits.
He also pointed out extinct volcanoes in the Bohemian coal fields as evidence that burning coal had produced them. This theory fits in well with religious belief in a great flood. Later, this theory came to be known as the Neptunist school.
Plutonist school
The opponents of the Neptunist school, like James Hutton, were known as Plutonists. Later, Leopold Von Buch, a student of Werner, travelled extensively and observed that coal beds have no relation with volcanoes. Finally, he broke with his teacher and became a supporter of the Plutonist school. When science advanced, more accurate observations came from geoscientists with the help of scientific instruments.
TYPES OF LAVA
Based on Silica percentage, there are two main types of lava.The first one is basic lava or basaltic lava. Second, Acidic lava.
Basic lavas are hot, highly fluid and less viscous lava. They are dark-coloured, rich in Magnesium and Iron, but poor in silica. Due to their fluidity, they flow readily, spread quickly from the source quietly, and are not very explosive. The volcano results from basic lava forms a flattened shield or dome.
Acidic lava is highly viscous, light-coloured, low-density lava with a high melting point. It flows slowly and results in a steep-sided volcano. The explosion is loud, and volcanic pyroclasts are emitted. Sometimes, acidic lava forms a plug. Plugs can be noticed at the caters of some volcanoes, like Mount Pelee. Plugs or stocks are the largest intrusive bodies of basic rocks.
CARBONATE BASED MAGMA
Carbonate-based Magma is less viscous and is the rarest type of terrestrial Magma. This categorisation is based on carbonate and not on silica. This lava is molten baking soda and erupts primarily in a few young continental spreading areasโ for example, the East African Rift Valley.
The Oldoingo Lengai, or mountain of god, in the Gregory Rift Valley of Tanzania, is the only historically active carbonate volcano in East Africa.
Now, a question? The melting temperature of Calcite is 1339 degrees Celsius. Then how does it melt at 550 degrees Celsius, only half the temperature of erupting Basalts? The Alkali-rich carbonate minerals with minor amounts of Nepheline and Pyroxene require much less heat to melt and make carbonate lavas position at the cold end of the magma temperature scale.
LAVA FLOWS
The fluid basaltic lava flow is classified into two types and is known by Hawaiian names: smooth-surfaced flow type called pah-hoy-hoy and rough-surfaced ah-ah. The smooth surface sometimes resembles the twisted braids of ropes. The pah-hoy-hoy means “on which one can walk”. Even though both can erupt from the same volcano, Pah-hoy-hoy lava is hotter and more fluid than ah-ah lava. Another interesting fact is that pah-hoy-hoy can change into ah-lava flow, and the reverse or ah-ah lava to pah-hoy-hoy lava does not occur.
Other names are also used in different languages. For examples-
Icelanders used the terms Helluhran and Apalhraum to describe the smooth and rough flows. In Japan, the rough surface type is known as Marubi. In Mexico, the rough surface type is known as Malpaos. In the Greek language, the term is dermolith and aphrolith.
ACTIVE AND DORMANT VOLCANOS
Based on their eruptive history, volcanoes are classified into active, dormant, and extinct.
Active volcanoes have erupted within the past several hundred years and may erupt again.
Dormants are potentially active but have not displayed their wrath of eruption within thousands of years.
Extinct volcanoes have not erupted for tens of thousands of years, and volcanic activity is not likely again.
WHAT ARE THE FACTORS AFFECTING THE VISCOSITY OF LAVA?
Viscosity or internal resistance to flow determines the mobility of Magma or lava. The more viscous fluid gives more resistance to flow.
The following factors influence viscosity of lava. High temperature makes lava less viscous. As the lava cools, the viscosity increases and an increase in viscosity gives greater resistance to flow. The resistance to flow is created due to strong internal resistance. The viscosity of lava is directly related to silica content; with an increase in silica content, viscosity increases. Silica restricts the flow of Magma or lava because, early in the crystallisation process, silicate tetrahedral structures start to link together into long chains. Water content can decrease the viscosity.
ANATOMY OF A VOLCANO
Before explaining the general anatomy of a volcano cone, we will examine the three major types of volcano cones. “shield“, “Cinder“, and “Composite”. Accumulation of fluid basaltic lava creates a shield volcano, which is a broad, slightly domed structure. The low viscosity of basaltic lava helps it to flow without any hindrance. It comes out from low-pressure situations, resulting in less violent explosions. The domed structure resembles a warrior’s shield. Due to continuous eruption, this structure can become heavy, resulting in crust bends. Hawaiian islands are an example.
Mauna Loa is considered the largest shield volcano on Earth, constructed by adding thin basaltic lava flows averaging a few meters thick intermixed with relatively small amounts of pyroclastic ejected materials. This volcano extends from its base on the Pacific floor and reaches over nine kilometres in height. Have you noticed the height !? Yes, it exceeds the height of Mount Everest, but we didn’t consider the portion in water. So Mount Everest got the title.
Ejected lava fragments from lava fountains begin to harden in flight to produce a cone-shaped hill or vesicular rock. These ejected pyroclastic fragment’s size ranges from fine ash to bombs exceeding one meter in diameter. Cinder cones are steep-sided with a thirty to forty-degree slope. Usually symmetrical in shape, some cinder cones are elongated and higher in the final eruptive phase. Cerro Negro of Nicaragua is an example.
Large, near-symmetrical volcano structures formed as the result of Felsic magma flow are known as composite volcanoes or strato volcanoes. It comprises interlayered lava, pyroclastic, tephra, and mud flows. These volcanoes also contain intrusions like dikes and sills. It erupts over long periods, which is the reason for their large size, shape and internal structure. The highest mountain in Africa, Mount Kilimanjaro in Tanzania, is an example. This mountain is located along the East African rift.
The Magma of the stratovolcano is highly viscous and gassy. Sometimes, it often blocks or plugs the vents, leading to an increase in pressure and resulting in a violent explosion. Another important composite volcano is Mount Fuji, near Tokyo. It is one of the most scenic and sacred composite volcanoes. During the last eruption in 1707, it ejected about eight hundred into ten, raised to six-meter cube of tephra, and statistically, mount Fuji is overdue for an explosion.
Every active volcano contains a vent or surface opening through which the hot lava emerges. It is a circular conduit that helps Magma rise from a deep chamber to a vent.
How Magma rises through the crust from the Magma chamber.
When a rock gets melted, two significant changes will happen. First, there will be a 10 per cent increase in volume than the original volume of rock. Second, there will be a decrease in the density. The less-dense Magma will rise through the fracture of overlying rocks. It is the pressure that helps to form this fissure. This pressure alone can bring the deep Magma to the surface. Both Magnetic and Tectonic forces also influence the fissures. These forces act on crustal rocks and produce fractures or fissures.
Now, we will examine the factors that influence the rising Magma. The pressure created by the overlying rocks pushes Magma upward. This pressure exerted on Magma decreases as the Magma rises higher into the crust.
Mafic Magma created from the partial melting of the mantle will have less density when compared with the surrounding rocks. When raised and reached the crust, the density of this Magma may be greater than that of crustal rocks. This helps to form a Magma chamber within the crust.
When the Magma rises upward, the pressure may decrease, allowing gases like Carbon dioxide, Sulfur dioxide, and water vapour to form bubbles in it. So, the density of the Magma decreases, and the Magma rises faster.
Tectonic stress allows Magma to open steep fractures and helps Magma to rise through fractures.
The viscosity of Magma decides its movement, and viscosity is decided by its temperature, composition and crystal contents. Under low temperatures, Magma will be very viscous. Under high temperatures, Magma will have low viscosity.
Felsic Magma has high silicon and oxygen content. This results in long silicate chains, which makes the Magma very viscous. The silicon and oxygen tetrahedra will link into long silicate chains. These chains are responsible for viscosity. When the Magma gets cooled, crystals will form. The crystals in the flowing Magma get in each other’s path and restrict the flow of Magma. Magama with few crystals will be less dense.
THE ORDER OF MAGAMA CRYSTALLISATION
When Magma reaches the surface, it transfers thermal energy through conduction and radiation to the atmosphere and the underlying surface. Rocks and minerals melt at different temperatures.
Felsic minerals melt before mafic minerals, and mafic minerals crystalise before felsic minerals. That means minerals crystallise in the opposite order from which they melt. The idealised sequence of mineral crystallisation is called Bowen’s reaction series. It describes the temperature at which minerals crystallise when cooled or melt when heated.
Mafic minerals, like Olivine, are the first to solidify from a mafic Magma. Since felsic lava lacks sufficient Magnesium, typically, minerals like Olivine do not crystalise from a felsic Magma.
Amphibole and Botite crystalise at temperatures lower than Olivine and Pyroxene but before most of the felsic minerals. Plagioclase feldspar with less calcium crystallise at lower temperatures. Felsic minerals like quartz and Muscovite crystalise at low temperatures. Minerals that crystalise late in the sequence will grow around the preexisting crystals. So, they grow as irregular, poorly defined crystals.
VOLCANIC AND PLUTONIC LANDFORMS
While travelling towards the Earth’s surface, Magma may cool and solidify within the Earth’s crust. The rocks formed in this manner are called Plutonic rocks. Plutonic rocks result in intrusive landforms. If lava gets solidified in the Earth’s crust, then it is called an extrusive landform. Rocks formed by either plutonic or volcanic activity are called igneous rocks. Batholiths or Bosses or Plutons are vast masses of igneous rocks, usually granitic in composition, that rise to the surface over millions of years through diapirs or hot plumes of rock ascending through cooler and denser country rock. We can notice batholiths on Kinabalu Mountain on the islands of Borneo.
TYPES OF VOLCANO AND LACROIX CLASSIFICATION
Professor Giuseppe Mercalli tried to distinguish between effusive eruption, explosive eruption and mixed eruption. He made this classification based on his observation of two well-known Italian volcanoes- “Stroboli” and “Vulcano“. Later, French mineralogist Alfred Lacroix created a detailed classification. He designated five types of eruptions and took four names of volcanoes to represent four types. They are Hawaiian, Strombolian, Peleean, and Vulcanian, and the fifth one is a mixed type. While creating this classification, he considered various factors like relative effusiveness, types of ejecta, Vents, and patterns of eruption.
Hawaiian-type means effusive eruptions of lava with little or no explosive activity apart from lava fountaining. The lava is primarily basaltic and originates at fissure vents. Associated with the building of shield volcanoes and flood-basalt plains.
Strombolian-type means moderately explosive eruptions producing cinder, bombs and ash, which are intrinsically incandescent as they leave the vent as they leave the vent. This type shows periodic blasts due to enormous gas bubbles in the vent.
Vulcanian-type means moderate to violent eruption of recently solidified lava. This is associated with the clearing of conduits, often plugged with domes.
Peleean-type means varied explosive activity, including the formation and destruction of domes and spines and the generation of destructive pyroclastic flows, termed “nuees ardentes“.
The mixed type has not survived the test of time. Due to the lack of descriptive specificity, geologists do not recognise this type.
Saucer-shaper igneous intrusions are called lopolith. They are vast and layered intrusions of basic rocks, typically of gabbro-type composition. For example, in Tasmania, dolerite magma intruded falt Permian and Triassic sediments, lifting them as the roofs.
VOLCANIC EXPLOSIVITY INDEX
You may be aware of the Ritcher scale used to measure seismic activity. Similarly, the volcanic explosive index is a scale to rack the strength or power of a volcanic eruption. This scale ranges from zero to eight with increasing intensity.
The factors that determine the number are the volume, height of the eruptive column, and duration of the eruption. Certain types of magma and volcano types are, more commonly, associated with different volcanic explosive indexes. For example, Basaltic fissure and shield volcanoes almost exclusively erupt with volcanic explosive indexes of zero or one. Strombolian erupts within a range of one and two or between small and moderate.